The 7 most common types of concrete damage and how to recognise them early on
Concrete is indispensable in industrial plants - from supporting structures and foundations to chimneys and Silos. However, like all materials, concrete is susceptible to damage that can affect its load-bearing capacity and service life. This article looks at the seven most common types of concrete damage in industrial environments: from mechanical cracking and spalling to chemical influences such as carbonation and chloride attack and biological damage caused by algae growth. Find out how modern Inspection methods help to recognise this damage at an early stage and how preventive measures can reduce maintenance costs and increase safety.
Introduction: Why concrete inspection is essential in industrial plants
Concrete forms the basis of many industrial structures - from supporting pillars to foundations to Storage tanks and silos. In heavy industry and power generation in particular, concrete structures are exposed to high loads and must be inspected regularly in order to detect damage at an early stage. Plant operators can thus increase safety and minimise unplanned downtime.
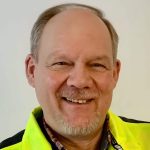
1. cracking due to mechanical loads
Cracks caused by tensile and shear forces: bending, shear and settlement cracks
Mechanical loads are one of the most common causes of cracking in concrete. These cracks - such as bending cracks (perpendicular to the component axis) and shear cracks (at a 45-degree angle) - occur when strength limits are exceeded and are often the first signs of structural weakness. As cracks allow moisture and pollutants to penetrate the structure, the risk of further damage increases. Corrosion.
Basic mechanisms of crack formation |
|
Classification of cracks according to load type |
|
Assessment of crack widths |
|
Assessment of the risk of cracking |
|
Preventive measures |
|
Monitoring and documentation |
|
2. chipping on edges and surfaces
Surface damage due to freeze-thaw cycles and mechanical effects
Spalling can occur due to mechanical stress or freeze-thaw cycles and expose the underlying reinforcement. Such damage is to be expected, especially in areas with high traffic or strong temperature fluctuations, and should be treated in good time to protect the steel reinforcement from corrosion.
Definition and appearance |
|
Types of damage |
|
Causes of spalling |
|
Damage analysis and assessment |
|
Repair measures |
|
Quality assurance |
|
Economic aspects |
|
3. carbonation: loss of corrosion protection
Chemical reaction caused by CO₂ - threat to the reinforcement
Carbonation is a slow process in which CO₂ from the air reacts with the concrete and lowers the pH value. This attacks the protective layer around the steel reinforcement, which can lead to corrosion. A simple phenolphthalein test can be used to visualise carbonation zones.
Basics of carbonation |
|
Effects on the reinforcement protection |
|
Factors influencing carbonation |
|
Measurement and monitoring |
|
Protective measures |
|
Repair |
|
Quality assurance |
|
4. chloride attack and pitting corrosion
Aggressive influences in road salt and coastal environments
In multi-storey car parks, bridges or facilities near the coast, chlorides can penetrate the concrete and cause pitting corrosion. This type of corrosion is localised and can weaken structural components abruptly. Regular inspection of such exposed structures is essential.
Basics of the chloride attack |
|
Corrosion mechanisms |
|
Damage mechanisms |
|
Examination and diagnosis |
|
Preventive measures |
|
Repair |
|
Monitoring and maintenance |
|
5. freeze-thaw damage
Repeated freeze-thaw cycles lead to structural loosening
In cold climates, water penetrates the pores of the concrete and freezes, damaging the structure and causing spalling. De-icing salts can accelerate this process. Regular inspection is advisable, especially in free-standing structures or unprotected areas of the system.
Basic damage mechanisms |
|
Factors influencing frost damage |
|
Typical damage patterns |
|
Reinforcing factors |
|
Examination and diagnosis |
|
Preventive measures |
|
6. sulphate attack and internal blasting
Reaction with sulphate-containing water - frequently in Industrial plants and wastewater structures
Sulphates from certain industrial wastewater or natural sources can lead to chemical reactions in concrete, known as ettringite formation. This process can crack the concrete structure from the inside and impair its stability. Such damage often occurs in systems that come into contact with sulphate-containing water.
Basics of sulphate attack |
|
Damage mechanisms |
|
7. biological damage caused by algae and moss
Damp concrete surfaces offer ideal conditions for growth
Algae and moss can thrive on damp concrete surfaces and contribute to long-term damage to the surface. In shaded areas or with poor drainage, biological growth can accumulate, increasing the moisture load on the concrete and weakening the surface.
Basics of biological fouling |
|
Damage mechanisms |
|
Damage patterns |
|
Risk factors and exposure |
|
Examination and diagnosis |
|
Preventive measures |
|
Cleaning and refurbishment |
|
Maintenance and servicing |
|
Modern inspection methods: Drones and sensor technology
Efficient inspection of hard-to-reach areas
Nowadays, modern inspection methods such as drones and sensors make it much easier to monitor concrete structures. Drones with high-resolution cameras, Kameras, Thermography and LiDAR can provide detailed images and models of areas that are difficult to access. This data can be used to systematically map and analyse damage. Ultrasonic sensors also make it possible to measure the thickness of the concrete in order to detect cavities or weak points at an early stage.
Preventive maintenance: a must for system operators
Cost reduction through regular inspections and preventive measures
Regular inspections and a preventive maintenance strategy are a worthwhile investment for operators. Recognising damage at an early stage and rectifying it in a targeted manner can prevent costly and time-consuming repairs and extend the service life of structures. A preventive maintenance strategy not only minimises costs, but also increases operational safety and avoids unplanned downtime.
Conclusion
Concrete is a robust building material, but it is susceptible to certain types of damage that can affect its service life and load-bearing capacity. With modern inspection techniques and a well thought-out maintenance strategy, plant operators can minimise the risk of damage and ensure the long-term safety of their structures. Regular inspections are the key to optimising maintenance and increasing efficiency in the industry.
Ensure the longevity of your concrete structures - let us work together to recognise and rectify damage at an early stage!
- We look forward to your enquiry and will respond promptly!
Frequently asked questions
Drones make it possible to quickly survey concrete surfaces that are difficult to access, such as elevated bridge areas or concrete façades. This allows inspections to be carried out more quickly, reducing downtime and labour costs.
Drones eliminate the need for inspectors to climb onto scaffolding or into dangerous areas such as high silos or narrow shafts. This improves safety by minimising risks for inspectors.
Modern drones are equipped with high-resolution cameras and special sensors that precisely document tiny cracks, spalling and surface damage on concrete surfaces and record them for analysis.
Underwater drones are ideal for concrete structures that are partially or completely under water such as bridge piers, harbour walls and dams. They enable inspection in difficult water areas without the need for time-consuming preparations such as lowering divers.
Drones capture detailed data that makes signs of damage such as cracks, corrosion and carbonisation visible. This allows damage to be recognised at an early stage and targeted measures to be taken before these problems become cost-intensive.
Using high-resolution photos and 3D models, drones can precisely depict surface conditions and structural deficiencies in concrete structures, making it easier to assess weak points such as cracks or changes in volume.
The data captured by drones can be used to create documentation, damage mapping and historical comparisons. This allows the progress of damage to be tracked and assessed over time.
Yes, drones can capture large areas such as tunnels and multi-storey car parks in a very short time and provide precise data for damage analysis and maintenance planning. This saves an enormous amount of time compared to manual inspections.
Drones with thermographic or LiDAR sensors can visualise temperature fluctuations and cracks caused by freeze-thaw cycles. This allows damage to be recognised at an early stage before it worsens.
By eliminating the need for expensive preparations such as scaffolding and cranes Drone inspections costs considerably. They can also be carried out more quickly, which also reduces indirect costs that could arise due to downtime.